Influence of soil texture on pollutants
- Abigail Clare
- Oct 4, 2021
- 10 min read
By E. Dring
Contaminated soils can be toxic for plants, animals, and humans. The texture of the soil can affect a pollutant’s mobility and bioavailability. Bioremediation methods can exploit different soil textures. But what is a soil texture?
Soil texture
The texture of soil was established by the pedogenic (soil-forming) processes of parent material, which can sometimes change over time with further weathering. Soils can be divided into twelve soil textures, characterised by the composition of sand, silt and clay, see Figure 1 (Osman, 2012).
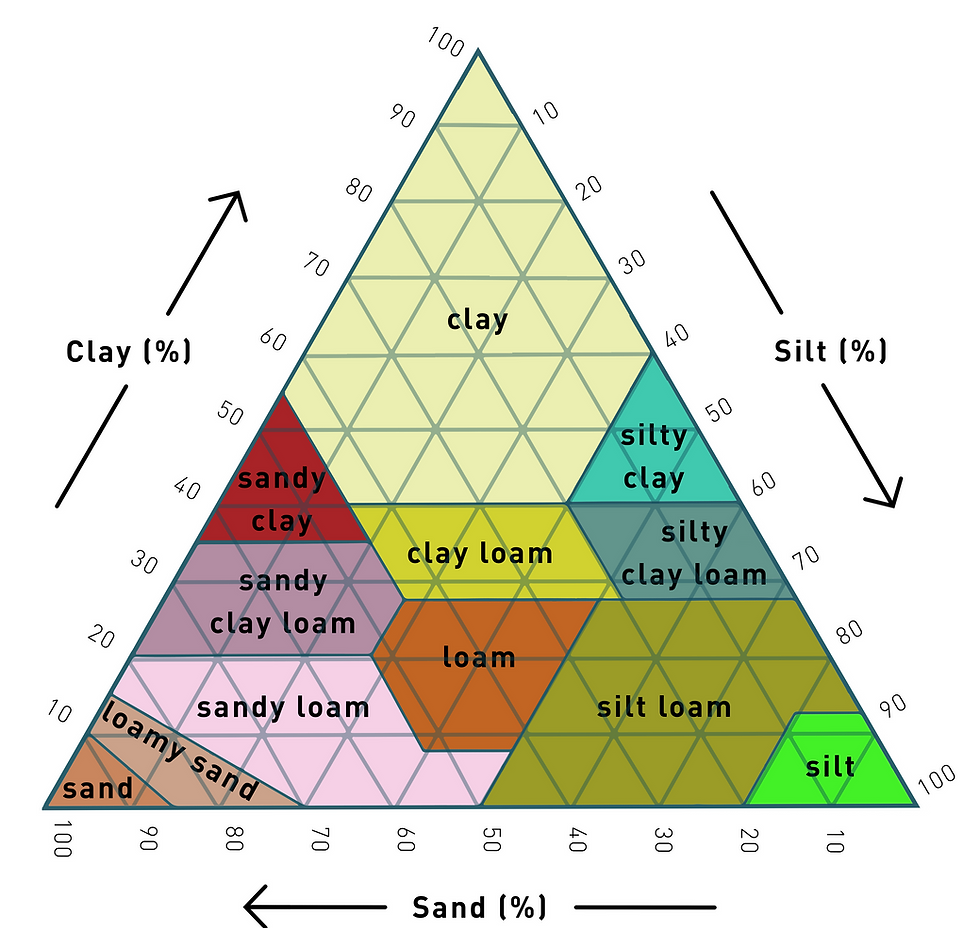
Figure 1: Soil texture triangle (adapted from Osman, 2012)
Sandy soils contain coarse particles (0.05-2.00 mm) with a large proportion of macropores (Osman, 2012). The pores allow for good water drainage; however, this can result in the leaching of nutrients into groundwater.
Silt soils are found in between sandy and clay particle sizes (0.002 and 0.05 mm). Silt is often found as a soil mixed with the other types (mentioned above and below) or is found as a suspension in water. Formed through the precipitation of sediments out of water they are often found under water bodies, e.g., seas and rivers. Silts retain water more than sands and are thus prone to holding pollutants. Silts do not contain as much organic content as clays.
Clay soils consist of fine particles (<0.002 mm) with a large proportion of micropores. They have a good water holding capacity, though are prone to waterlogging (Osman, 2012). Fertility tends to be higher in fine grained soil. This is due to a higher soil organic matter content, cation exchange capacity and thus, more available nitrogen for plants. The number of microorganisms is also higher in finer soils (Osman, 2012).
Peat soil is formed when organic materials are deposited at a faster rate than what microorganisms can completely decompose it (Rich, 2015). Peat soils have a high water retention capacity, which maintains saturated conditions and poor aeration (Bell, 2005). Peat use is considered against environmental sustainability as peat is sequestered carbon that can continue to sequester carbon if left in the ground. The use of peat enhances climate change as it releases biogenic carbon that is en route to becoming fossil carbon.
Soil properties
pH
Podzol and Alisol are examples of acid soil groups (FAO, N.D.). When soils are acidic, hydrogen competition is high (Makovníková et al., 2007) – that is hydrogen ions are so common that the material in the soils are greedy in trying to get the hydrogens. Metals such as aluminium can increase the acidity of soil and conversely acid also causes an increase in the number of metals (metals are more soluble in acids). Molybdenum can help to neutralise acidic soils (FAO, N.D.).
In alkaline soils, hydrolysis of cations with a low susceptibility is higher than when compared to acidic soils (Makovníková et al., 2007). Hydrogen bonds between clay minerals and organic compounds are broken, in turn, increasing the mineralisation of chemical elements (Neina, 2019). Mineralisation is one of the key features of soil, i.e., the conversion of materials that are unavailable to plants, to become bioavailable nutrients. Acid or alkaline mineralisation of elements greatly benefit plants.
Soil organic matter
Organic matter in clay soils is isolated in the smaller soil pores, or are associated with clay particles themselves, inside a sand/clay substrate. According to Hassink et al. (1993), soil organic matter associated with clays are found to be more decomposed therefore, have a lower carbon to nitrogen (C:N) ratio – as the carbon is lower. Hamarashid et al., (2010) found that soils containing a higher percentage of clay had a higher respiration rate of CO2 – indicating decomposition of carbon. Higher organic matter content being decomposed will respire more (McLauchlan, 2006).
Franzluebbers et al., (1996) found that nutrients were more readily available to plants in sandier soils, because soils with a lower clay content have a higher mineralisation of carbon and nitrogen. Bacteria in sandy soils have a higher mineralisation rate of nitrogen compared to loam and clay soils. However, they face greater grazing pressures from bacterivorous nematodes (Hassink et al., 1993).
Cation exchange capacity, CEC
A soils cation exchange capacity (CEC) plays an important role in retaining ions for plant nutrition (Hatten and Liles, 2019). Due to clay soils having a high specific surface area and a high organic matter content, they tend to have a high cation exchange capacity (Figure 2). Compost has been found to increase the cation exchange capacity of soil with a high clay content (above 22 %), which have a higher initial exchange capacity (Duong et al., 2012). Therefore, clay soils are often more fertile.

Figure 2: Sandy/clay soil with clay at 40 cm illustrating the effect of organic matter at the clay-line, and the increase in CEC (SOA, N.D.)
The opposite is true if the soil is nutrified using inorganic fertilisers. Inorganic fertilisers lower the soil pH, lowering the CEC and starving the plants of cations such as K+, Mg2+, and Ca2+. Adding lime to soils can increase the CEC again, so the mineralisation of soils is the key to plant nutrition.
Temperature
A soil’s temperature can alter the germination of plants. It has been found that heat capacity varies with soil texture, with sandy soils having a lower heat capacity (Abu-Hamdeh, 2003). Soils that have a low ability to heat is particularly useful in places where the soil is exposed to intense sunshine. Soil thermal conductivity is another useful attribute to keeping soils cool. If the soil conducts heat well, the hot surface heat will travel into cooler lower sediments allowing the soil surface to cool, encouraging plant germination.
The particle size of the soil is ultimately the determining factor. Soils with a smaller particle size have a higher surface area to volume ratio. Clays therefore have a greater surface area which means it can trap more heat and it will have greater surface contact with other fine particles.
Metals
When soil is contaminated with a transition metal, oxidising chemical reactions take place. Depending on the properties of chemicals produced, such as mobility and bioavailability, effects of the metal may differ (Wuana and Okiemen, 2011). Oxidation will change the organic matter in the soil into nutrients that are no longer bioavailable, e.g., the oxidation of ammonia will result in nitrates and nitrites (called nitrification) – which is good for plants. However, as metals are commonly associated with lower pH, the nitrification is lowered as the pH is lowered which is not good for plants. Soil organic bioavailable carbon (and nitrogen) has been found to decrease when soils are polluted with metals, in turn, effecting plant growth (Zhou et al., 2016). The uptake of metals by plants increases when other micronutrient concentrations are too low (Fijałkowski et al., 2012).
Metals of poor mobility include chromium and nickel. Cadmium, zinc and molybdenum have a high mobility, which increases when soil aeration is reduced (Fijałkowski et al., 2012). The solubility of cadmium has been found to be greater in acid forest soils compared to agricultural soils (Richards et al., 2000). The toxicity threshold of cadmium is dependent on a soils texture. The activity of microorganisms and enzymes is reduced when exposed to cadmium, this reduction is greater in sandy loam soils compared to clay loam and loam (Hassan et al., 2013).
Soil properties effect the bioavailability of metals. Soils with a large clay content have a greater cation sorption ability, compared to soils with a lower clay percentage. Fijałkowski et al., 2012) states that if metals are absorbed by organic matter, then less is available for plants.
Soil organic bioavailable carbon (and nitrogen) has been found to decrease when soils are polluted with metals, in turn, effecting plant growth (Zhou et al., 2016). The uptake of metals by plants increases when micronutrient concentrations are too low (Fijałkowski et al., 2012).
When soils have a pH value greater than 6.5, dissolved organic matter becomes mineralised, in turn, increasing the mobility of metal contaminants (Richards et al., 2000). On the other hand, highly acidic soils have also been found to increase the mobilisation of metals (Fijałkowski et al., 2012), but the organic material does not mineralise leading to largely just uptake of the metals (increasing the concentration of metals in plant tissues). High concentrations of transition metals in plants (that can oxidise plant tissues) can be lethal to plants and are certainly unhealthy to eaters of that plant.
Biodegradability
Some transition metals are found to alter the structure of microorganisms, inhibiting their ability to biodegrade organic matter. Several methods can be implemented to increase biodegradation. For example, the addition of metal-resistant bacteria and clay minerals to soil can limit the bioavailability of metals (Sandrin, and Maier, 2003).
Soil characteristics are also affected by oil pollution. The water-holding capacity of soil has been found to decrease when polluted with oil (Wei et al., 2019). Petroleum hydrocarbons can be decomposed if concentrations are less than 5% (Galitskaya et al., 2021). The most favourable soil pH for the degradation of oil pollution is between 6.5 and 8 (Neina, 2019). Increasing oil pollution up to 25% alters the populations of microorganisms, favouring oil-degrading bacteria (Galitskaya et al., 2021). Leathers containing high extractable or total mineral oils can be a source of oil contaminants in soil.
Bioremediation
Contaminants can be transformed during bioremediation, and soil properties may change. Toxic elements are reduced; therefore, the diversity of soil organisms should increase (Haimi, 2000).
Compared to clay loam,petrochemicals are found to be more easily removed in sand through the extraction of pressurised liquid. This is also due to the oil adhesion onto the fine clay particles (Abdel-Moghny, et al., 2012). Surfactant remediation has been found less efficient in fine soils, due to clay sorption. Hydraulic flow is affected by the interactions between surfactant and clay. Impaired permeability can reduce the remediation efficiency of hydrocarbons (Lee et al., 2002). Haghallahi et al., (2016) similarly found that bioremediation was more effective for sandy soils compared to clay, which may also be caused by less oxygen being available for microorganisms in fine soils.
An effective method for the removal of chlorinated hydrocarbons in soil is through a mechanical soil aeration method, which effectively converts the pollutant to vapour for transferring. Aeration converts the chlorinated into an oxidised form making it more volatile. The efficiency has been found to decrease when soil is coarser (Ma et al., 2017) because of the larger pore size and easier aeration.
Conclusion
Soil textures are identified by there percentage of sand, silt, and clay. Clay soils are fine, through the intermediate silt, and into sandy soils which are coarser. Soils have a variety of properties which influence how they respond to pollutants.
Fine-textured soil with high concentrations of organic matter and micronutrients are generally more able to trap metals. Small concentrations of petrochemical pollutants can be degraded when soil has a neutral pH. Sandy soils are favourable when using pressurised liquid remediation to remove hydrocarbons. However, mechanical soil aeration methods are suitable for fine-textured soil.
References:
Abdel-Moghny, T., Mohamed, R.S., El-Sayed, E., Mohammed Aly, S. and Snousy, M.G., 2012. Effect of soil texture on remediation of hydrocarbons-contaminated soil at El-Minia district, Upper Egypt. International Scholarly Research Notices.
Abu-Hamdeh, N.H., 2003. Thermal properties of soils as affected by density and water content. Biosystems engineering, 86(1), pp.97-102.
Bell, F.G., 2005. ENGINEERING GEOLOGY | Problematic Soils, Encyclopedia of Geology, 554-565.
Duong, T.T., Penfold, C. and Marschner, P., 2012. Differential effects of composts on properties of soils with different textures. Biology and Fertility of Soils, 48(6), pp.699-707.
FAO. No Date, N.D. Soils Portal. Available at: http://www.fao.org/soils-portal/soil-management/management-of-some-problem-soils/acid-soils/en/
Fijałkowski, K., Kacprzak, M., Grobelak, A. and Placek, A., 2012. The influence of selected soil parameters on the mobility of heavy metals in soils. Inżynieria i Ochrona środowiska, 15, 81-92.
Franzluebbers, A.J., Haney, R.L., Hons, F.M. and Zuberer, D.A., 1996. Active fractions of organic matter in soils with different texture. Soil Biology and Biochemistry, 28(10-11), 1367-1372.
Galitskaya, P., Biktasheva, L., Blagodatsky, S. and Selivanovskaya, S., 2021. Response of bacterial and fungal communities to high petroleum pollution in different soils. Scientific Reports, 11(1), 1-18.
Haghollahi, A., Fazaelipoor, M.H. and Schaffie, M., 2016. The effect of soil type on the bioremediation of petroleum contaminated soils. Journal of environmental management, 180, 197-201.
Haimi, J., 2000. Decomposer animals and bioremediation of soils. Environmental Pollution, 107(2), 233-238.
Hamarashid, N.H., Othman, M.A. and Hussain, M.A.H., 2010. Effects of soil texture on chemical compositions, microbial populations and carbon mineralization in soil. Egypt. J. Exp. Biol.(Bot.), 6(1), 59-64.
Hassan, W., Akmal, M., Muhammad, I., Younas, M., Zahaid, K.R. and Ali, F., 2013. Response of soil microbial biomass and enzymes activity to cadmium (Cd) toxicity under different soil textures and incubation times. Australian Journal of Crop Science, 7(5), 674-680.
Hassink, J., 1994. Effects of soil texture and grassland management on soil organic C and N and rates of C and N mineralization. Soil Biology and Biochemistry, 26(9), .1221-1231.
Hassink, J., Bouwman, L.A., Zwart, K.B., Bloem, J. and Brussaard, L., 1993. Relationships between soil texture, physical protection of organic matter, soil biota, and C and N mineralization in grassland soils. In Soil Structure/Soil Biota Interrelationships, 105-128.
Hatten, J. and Liles, G., 2019. A ‘healthy’balance–The role of physical and chemical properties in maintaining forest soil function in a changing world. In Developments in Soil Science, 36, 73-396.
Lee, D.H., Cody, R.D., Kim, D.J. and Choi, S., 2002. Effect of soil texture on surfactant-based remediation of hydrophobic organic-contaminated soil. Environment International, 27(8), 681-688.
Ma, Y., Shi, Y., Hou, D., Zhang, X., Chen, J., Wang, Z., Xu, Z., Li, F. and Du, X., 2017. Treatability of volatile chlorinated hydrocarbon-contaminated soils of different textures along a vertical profile by mechanical soil aeration: A laboratory test. Journal of Environmental Sciences, 54, 328-335.
Makovníková, J., Barancikova, G. and Pálka, B., 2007. Approach to the assessment of transport risk of inorganic pollutants based on the immobilisation capability of soil. Plant Soil and Environment, 53(8), p.365.
McLauchlan, K.K., 2006. Effects of soil texture on soil carbon and nitrogen dynamics after cessation of agriculture. Geoderma, 136(1-2), 289-299.
Neina, D., 2019. The role of soil pH in plant nutrition and soil remediation. Applied and Environmental Soil Science, 2019.
Osman, K.T., 2012. Soils: principles, properties and management. Springer Science & Business Media.
Rich, F. J., 2015. "Peat, Its Origins, Characteristics, and Geological Transformations." Coal and Peat Fires: A Global Perspective, Glenn B. Stracher, Anupma Prakash and Guillermo Rein (Ed.), 4: 13-38: Elsevier Ltd.
Richards, B.K., Steenhuis, T.S., Peverly, J.H. and McBride, M.B., 2000. Effect of sludge-processing mode, soil texture and soil pH on metal mobility in undisturbed soil columns under accelerated loading. Environmental pollution, 109(2), pp.327-346.
Sandrin, T.R. and Maier, R.M., 2003. Impact of metals on the biodegradation of organic pollutants. Environmental Health Perspectives, 111(8), 1093-1101.
SOA – Soilquality.org.au (N.D.) Fact sheet: Cations and cation exchange capacity. [Online]. Available at: https://soilquality.org.au/factsheets/cation-exchange-capacity. (accessed 26 September 2021).
Wuana, R.A. and Okieimen, F.E., 2011. Heavy metals in contaminated soils: a review of sources, chemistry, risks and best available strategies for remediation. International Scholarly Research Notices.
Zhou, T., Li, L., Zhang, X., Zheng, J., Zheng, J., Joseph, S. and Pan, G., 2016. Changes in organic carbon and nitrogen in soil with metal pollution by Cd, Cu, Pb and Zn: a meta‐analysis. European Journal of Soil Science, 67(2), pp.237-246.
Comments